
And so this nitrogen might wanna do what we call in organic chemistry a nucleophilic attack on this carbon right over here. And this carbon right over here, it's attached to two oxygens, oxygens are more electronegative. Well, nitrogen, as we said, has got this lone pair, it's electronegative. I'm not gonna do a formal reaction mechanism, but really get a sense of what's going on. But whenever I see a reaction like this, it's somewhat satisfying to just be able to do the counting and say, "All right, this is gonna bond "with that, we see the bond right over there, "and I'm gonna let go of an oxygen and two hydrogens, "which net net equals H2O, equals a water molecule." But how can we actually imagine this happening? Can we push the electrons around? Can we do a little bit of high-level organic chemistry to think about how this happens? And that's what I wanna do here. We saw this type of reaction when we were putting glucoses together, when we were forming carbohydrates. condensation reaction or dehydration synthesis. And because you have the release of this water molecule, this type of reaction, and we've seen it many other times with other types of molecules, we call this a condensation reaction, or a dehydration synthesis. So this reaction, you end up with the nitrogen being attached to this carbon, and a release of a water molecule. that's let go from both of these amino acids. So this lone pair goes to this carbonyl carbon, forms a bond, and then this hydrogen, this hydrogen, and this oxygen could be used net net to form a water molecule. And a very high-level overview of this reaction is that this nitrogen uses its lone pair to form a bond with this carbonyl carbon right over here. That would be the smallest possible peptide, but then you could keep adding amino acids and form polypeptides. And so, how do you take these two amino acids and form a dipeptide like this? A dipeptide would have two amino acids. And what we're gonna concern ourselves with in this video is, how do you take two amino acids and form a peptide out of them? And just as a reminder, a peptide is nothing more. And that alpha carbon is gonna be bonded to a hydrogen and some type of a side chain, and we're just gonna call this side chain R1, and then we're gonna call this side chain R2. And in between we have a carbon, and we call that the alpha carbon. We have a carboxyl group right over here. We have an amino group right over here that gives us the amino and amino acid. We recognize the telltale signs of an amino acid. So I've got two arbitrary amino acids here. Hopefully I am not misleading anyone, I'm just assuming this is the case. Water has no net charge to begin with, just an unequal distribution of electrons across the molecule. But this isn't an ionic compound! Rather we can look at it as an ion (Acting as a cation and anion simultaneously) with two opposite charges that cancel out, making it a zwitterion (hybrid ion)! Just like any ionic compound, the charges are opposite and equal in magnitude and therefore cancel out. Unlike the water molecule, these net charges are independent of each other. On the other side, we have a carboxyl group which let go of a proton therefore gaining a -1 net charge (negative). In the case of the amino acids shown in the video above, we have an amino group which gained a proton from the solution and its net charge became + 1 (positive). At this point, the molecule is polar, additionally there are no formal charges. The oxygen atom "Hogs" the electrons from the covalent bond creating a partially negative side in the molecule while the other side becomes partially positive.
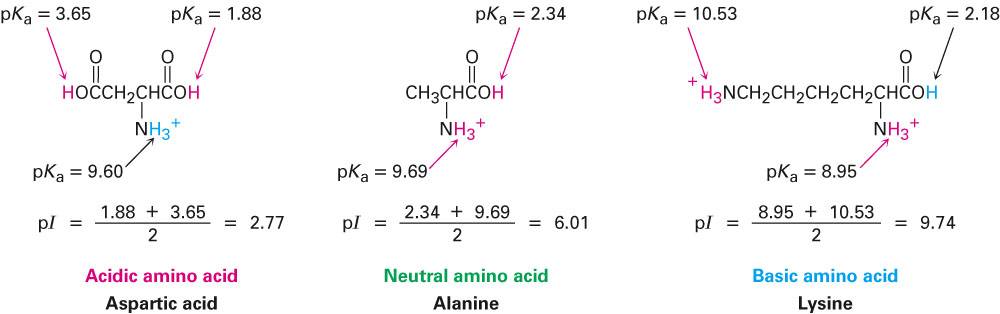
I assume your textbook is rounding.I thought of that too, but my guess would be no.Ī water molecule is considered polar because of electronegativity differences between the oxygen atom and the 2 hydrogen atoms. The average charge of methionine at pH 7 is simply the sum of these mole fractions, accounting for the charge of each species, and is approximately equal to -0.006109. To state it another way, ~99.9981% of the carboxyl groups are in the deprotonated (negative) state and ~99.3872% of the amino groups are in the protonated (positive) state. We can calculate this distribution and therefore the average charge of the species with the Henderson-Hasselbalch equation: You are right in your reasoning: at any pH for any titratable group, there is a distribution between the protonated and deprotonated species.
